Research Projects
Electrostatically defined nanodevices in bilayer graphene
(Chuyao Tong, Rebekka Garreis, Lisa Gächter, Wister Wei Huang, Max Ruckriegel, Hadrien Duprez, Christoph Adam, Jonas Gerber)
Due to carbon’s low atomic mass and naturally low nuclear spin concentrations, carbon-based devices have weak spin-orbit coupling and weak hyperfine interaction, making them promising platforms for hosting spin qubits. In bilayer graphene, due to the interlayer coupling, a displacement field applied perpendicular to the sheet induces a bandgap. Exploiting this bandgap opening using appropriate top- and back- gate electrodes, we can electrostatically confine charge carriers in our high-quality bilayer graphene samples, forming ambipolar single- and multi- quantum dot systems with tunable barriers [1,2,3]. We study the interesting physics of these quantum dots, such as their excited states and their magnetic field dependence [4,5], the Kondo effect [6], Pauli spin and valley blockade [7]. We can also integrate a charge detector into our devices [8] and investigate the full counting statistics of a single electron tunneling through the quantum dot, as well as performing single-shot spin readout to measure the spin relaxation time T1 [9]. We aim to advance towards graphene-based quantum computation. Moreover, we utilize the charge detector to perform entropy measurements of quantum systems and further investigate the degeneracy and underlying physics of the ground and excited states of the quantum dots.
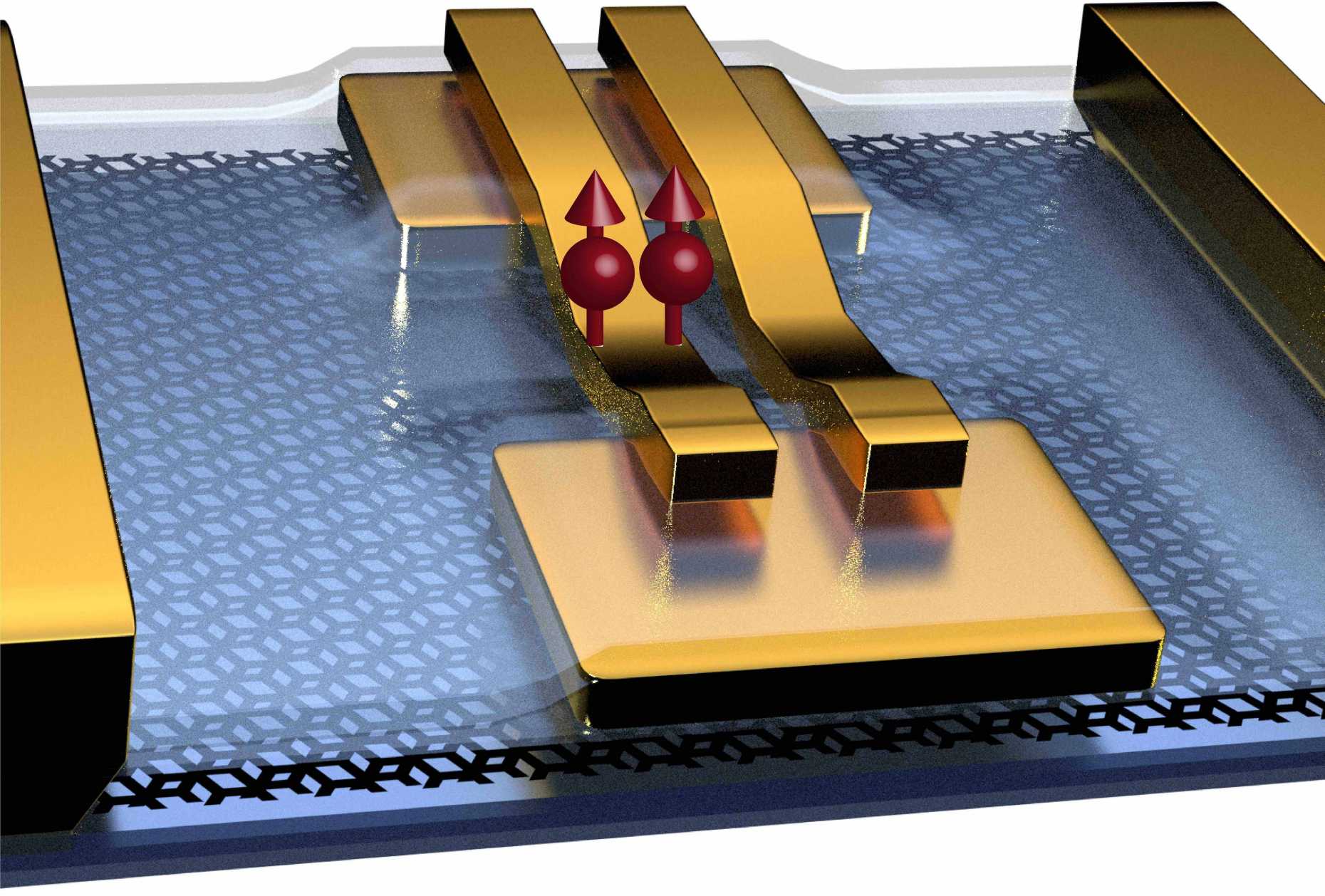
References:
[1] Eich, M. et al. Coupled Quantum Dots in Bilayer Graphene. Nano Lett. 18, 5042–5048 (2018).
[2] Tong, C. et al. Tunable Valley Splitting and Bipolar Operation in Graphene Quantum Dots. Nano Lett. 21, 2, 1068-1073 (2021).
[3] Garreis, R. et al. Shell Filling and Trigonal Warping in Graphene Quantum Dots. Phys. Rev. Lett. 126, 147703 (2021).
[4] Eich, M. et al. Spin and Valley States in Gate-Defined Bilayer Graphene Quantum Dots. Phys. Rev. X 8, 1–11 (2018).
[5] Kurzmann, A. et al. Excited States in Bilayer Graphene Quantum Dots. Phys. Rev. Lett. 123, 026803 (2019).
[6] Kurzmann, A. et al. Kondo effect and spin-orbit coupling in graphene quantum dots. Nat. Comm. 12, 6004 (2021).
[7] Tong, C. et al. Pauli Blockade of Tunable Two-Electron Spin and Valley States in Graphene Quantum Dots. Phys. Rev. Lett. 128, 067702 (2022).
[8] Kurzmann, A. et al. Charge Detection in Gate-Defined Bilayer Graphene Quantum Dots. Nano Lett. 19, 5216–5221 (2019).
[9] Gächter, L. M. and Garreis, R. et al. Single-Shot Spin Readout in Graphene Quantum Dots. PRX Quantum 3, 020343 (2022).
Electronic properties of transition metal dichalcogenides (TMDs)
(Michele Masseroni, Jonas Gerber)
Transition metal dichalcogenides (TMDs) are a family of two-dimensional materials with broad electronic properties ranging from semiconductors to semi-metal and superconductors. In our laboratory we focus on semiconductors (e.g. MoS2 and WSe2). We investigate the band structure of mono- [1], bi- [2], and trilayer [3,4] MoS2 by means of magnetotransport experiments. We further take advantage of the large band gap (1-2 eV) to define nanostructures, demonstrating gate-defined quantum point-contact [5], as well as quantum dots [3]. In our future research projects we exploit the intrinsic spin-orbit coupling of TMDs in graphene-based qunatum devices.
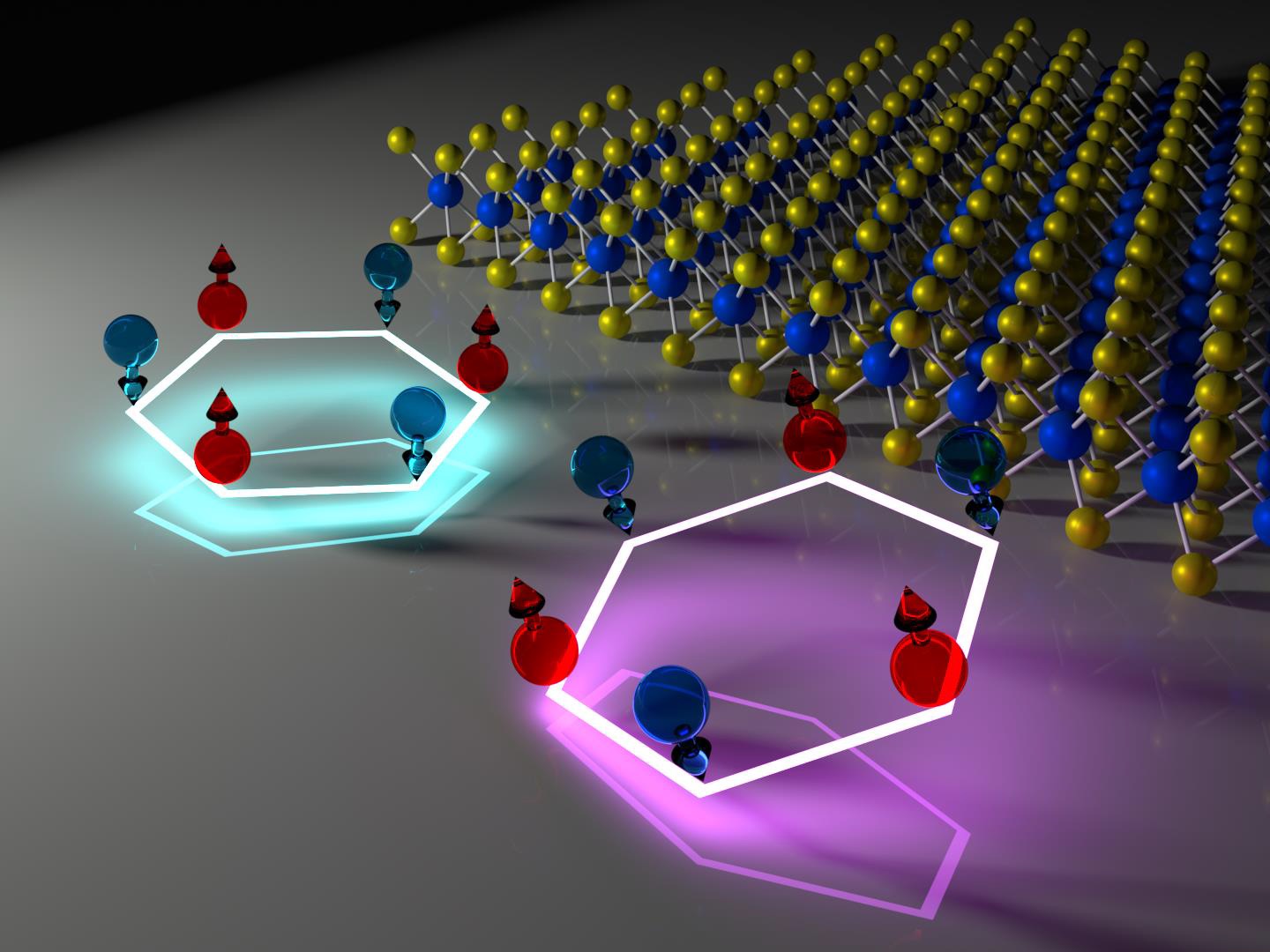
References:
[1] Pisoni, R. et al. Interactions and Magnetotransport through Spin-Valley Coupled Landau Levels in Monolayer MoS2. Phys. Rev. Lett. 121, 247701 (2018).
[2] Pisoni, R. et al. Absence of Interlayer Tunnel Coupling of K-Valley Electrons in Bilayer MoS2. Phys. Rev. Lett. 123, 117702 (2019).
[3] Pisoni, R. et al. Gate-Defined One-Dimensional Channel and Broken Symmetry States in MoS2 van der Waals Heterostructures. Nano Lett. 17, 5008-5011 (2017).
[4] Masseroni, M. et al. Electron Transport in Dual-Gated Three-Layer MoS2. Phys. Rev. Lett. 3, 023047 (2021).
[5] Pisoni, R. et al. Gate-Tunable Quantum Dot in a High-Quality Single Layer MoS2 van der Waals Heterostructure. Appl. Phys. Lett. 112, 123101 (2018).
Twisted 2D heterostructures
(Shuichi Iwakiri, Elias Portolés, Giulia Zheng, Marta Perego)
When two layers of graphene are twisted with respect to each other and superimposed, a moiré pattern appears. The characteristic lengthscale of the resulting system is thus that of the moiré pattern, orders of magnitude larger than the interatomic distance of a single graphene layer. Moreover, when the twist between layers corresponds to the so-called 'magic-angle' the conduction and valence bands of the system flatten, leading to a vanishing Fermi velocity. This results in a large Coulomb interaction energy compared to the kinetic energy of the electrons. At particular electronic densities, these interactions drive a transition to a superconducting phase.
We make use of the expertise of the group in device nanofabrication to harness such field-effect-tunable superconductivity. In particular, we focus on the mesoscopic superconducting effects exhbited by Josephson junctions [1] and superconducting quantum interference devices (SQUIDs) [2] in this material. Looking ahead, we engineer more complex devices allowing us to both probe the material and exploit its properties, aiming for applications in the field of superconducting electronics.
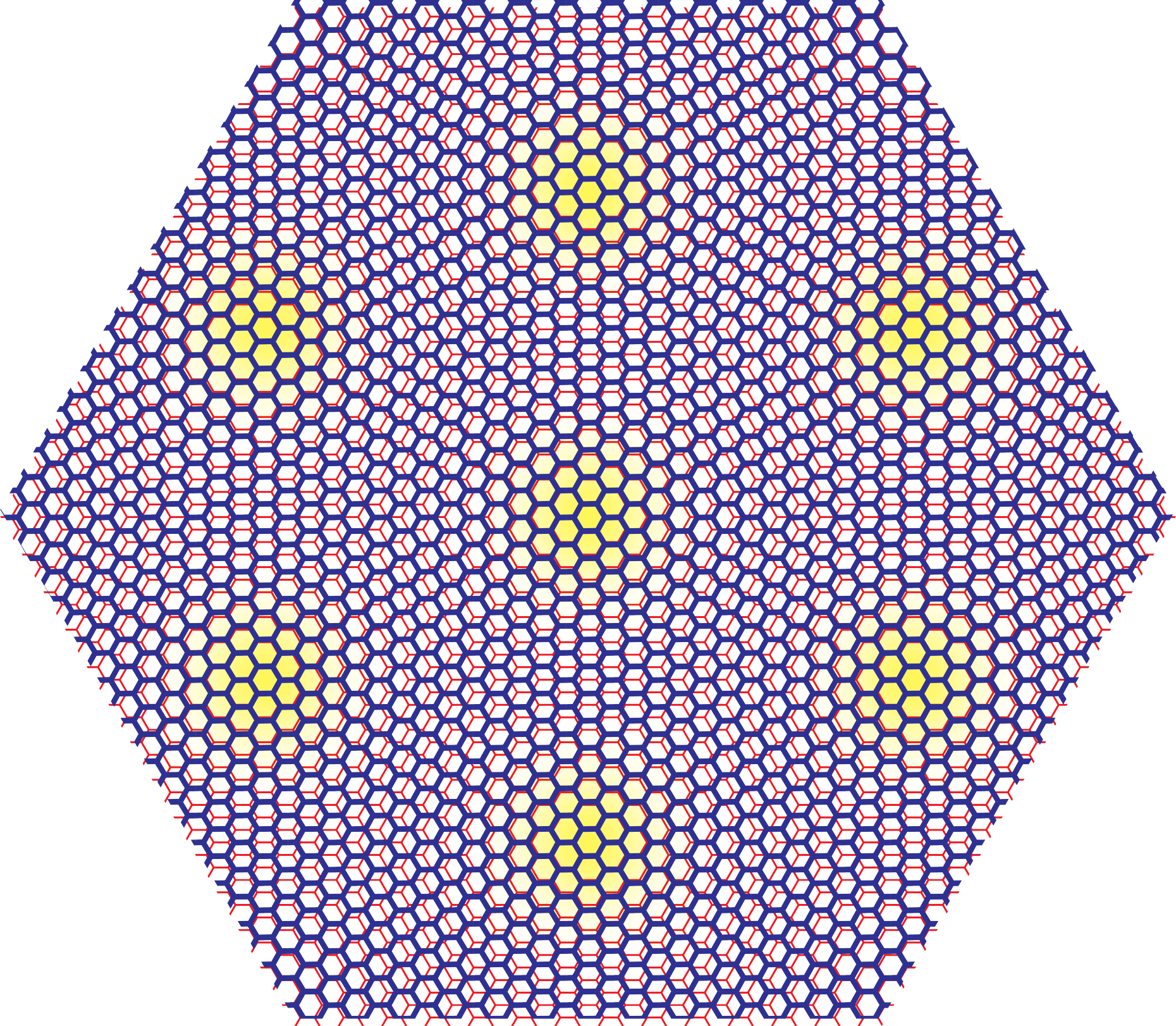
References:
[1] F. K. de Vries, et al., Nature Nano 16, 760 (2021).
[2] E. Portoles, et al., arXiv:2201.13276 (2022).
Fractional quantum Hall effect in confined geometries
(Marc Röösli, Giorgio Nicolí)
Two-dimensional charge-carrier systems subjected to strong magnetic fields display interesting quantum phenomena, such as the integer and fractional quantum Hall effects. Electron transport in this regime is dominated by one-dimensional, topologically protected edge channels, while the bulk remains insulating. Even though the present understanding is supported by extensive theoretical and experimental work, several questions remain open.
We perform electron transport experiments on 2D electron gases formed in in GaAs/AlGaAs heterostructures in the integer and fractional quantum Hall regime. Applying voltages to patterned top gate electrodes, we form nanostructures in the 2D electron gas with a typical device shown in Fig. 1. Experiments on weak backscattering in quantum point contacts have shown Luttinger liquid behavior of fractional quantum Hall states [1, 2]. Using 1µm-sized quantum dots we study tunneling phenomena between compressible stripes confined in the dot [3]. We find a continuous transition from a Coulomb dominated island to interferometer behavior. Changes in quasi-particle number manifest as phase-jumps in the interferometer signal and can potentially be used to infer additional information on their statistics. Additionally, we selectively transmit and reflect edge channels with gate-defined structure to create out of equilibrium situations relatively to their individual electrochemical potential [4]. In such a situation, we can measure the degree of equilibration reached by these channels relative to specific integer and fractional states.
Investigating fragile fractional states and improving fractional quasiparticle coherence requires low electronic temperatures. Currently reaching electronic temperatures below 6 mK, we emphasize improving low temperature measurement setups and techniques [5].
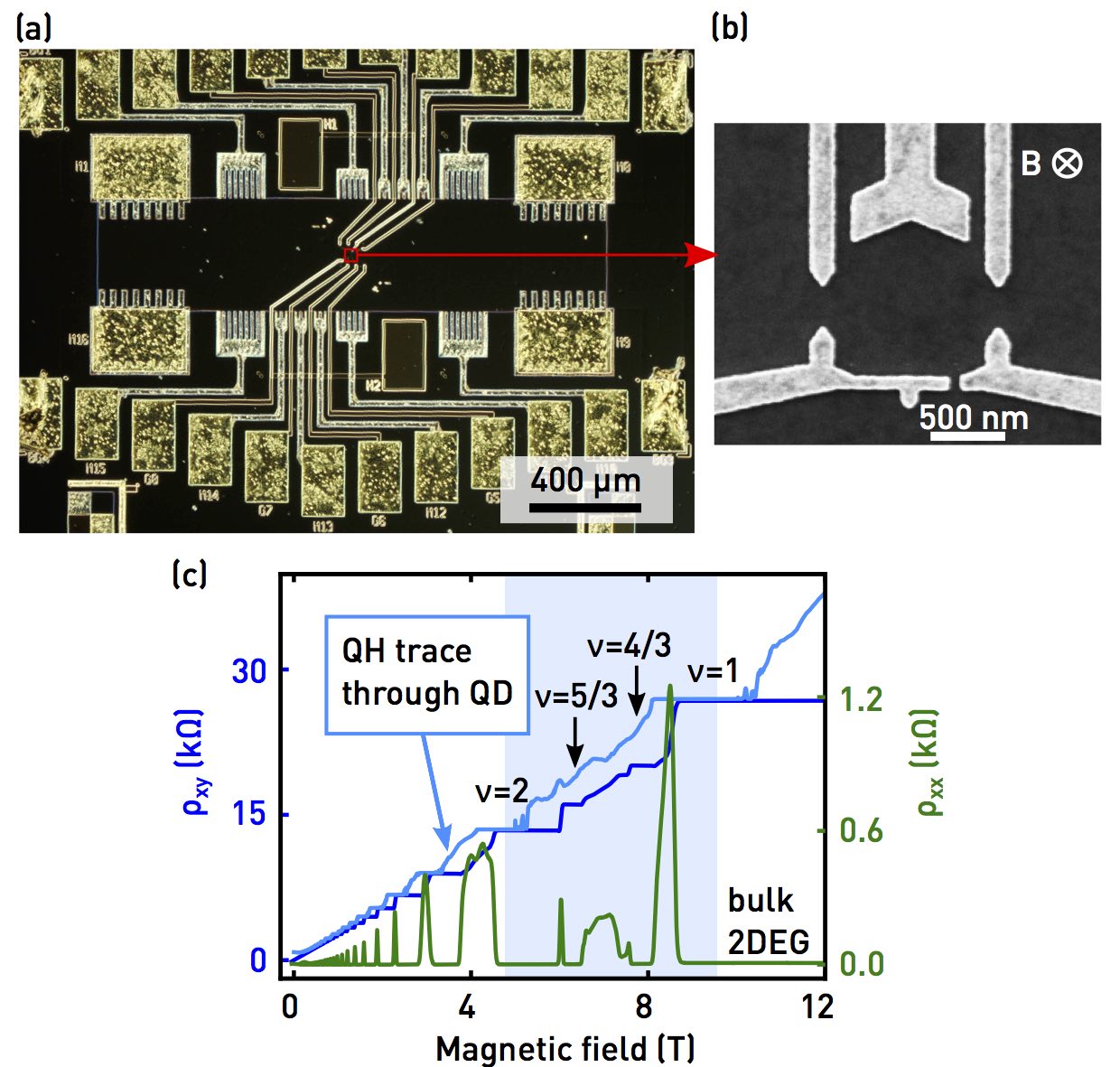
Fig 1. – (a) Optical microscope image of a typical sample. (b) Scanning electron micrograph of the section marked in (a) showing a 1µm-sized quantum dot. (c) Hall measurement: transversal and longitudinal resistance as a function of magnetic field. Quantized transversal resistance with corresponding vanishing longitudinal resistance is observed for integer and fractional quantum Hall states.
References:
[1] S. Baer, C. Rössler, T. Ihn, K. Ensslin, C. Reichl, and W. Wegscheider Phys. Rev. B 90, 075403 (2014)
[2] S. Hennel, P. Scheidegger, M. Kellermeier, A. Hofmann, T. Krähenmann, C. Reichl, W. Wegscheider, T. Ihn, and K. Ensslin, Phys. Rev. B 97, 245305 (2018)
[3] M. P. Röösli, L. Brem, B. Kratochwil, G. Nicolí, B. A. Braem, S. Hennel, P. Märki, M. Berl, C. Reichl, B. Rosenow, W. Wegscheider, K. Ensslin, T. Ihn, ArXiv:1910.12525 [Cond-Mat] (2019)
[4] R. J. Haug, Semicond. Sci. Technol 8, pp. 131 (1993)
[5] G. Nicolí, P. Märki, B. A. Bräm, M. P. Röösli, S. Hennel, A. Hofmann, C. Reichl, W. Wegscheider, T. Ihn, and K. Ensslin, Rev. Sci. Instrum 90, 113901 (2019)
Superconducting-semiconductor hybrid devices
(Benedikt Kratochwil, Lisa Gächter, Max Ruckriegel, Wister Huang)
In a joint collaboration with the Wallraff group we investigate different gate defined quantum dot qubits in GaAs and graphene nanostructures. To probe the qubit properties we use a circuit QED based approach. We capacitively couple a superconducting coplanar waveguide resonator to one of the quantum dot plunger gates to introduce coupling between the resonator photon and the qubit.
In Anna Stocklauser et al. [1] we showed strong coupling of a double quantum dot charge qubit to a single microwave photon residing in the resonator. A key ingredient for the success of this experiment was the use of a high impedance resonator, enhancing the coupling strength by approximately one order of magnitude compared to standard 50 Ω resonator designs. Employing similar techniques we recently showed strong spin photon coupling [2]. The spin qubit was implemented in a resonant exchange qubit which allows to couple the spin and charge degree of freedom of the qubit to enhance the coupling strength. In succession to these works we showed two qubit coupling for two charge qubits [3], charge and Transmon qubits [4] and spin and Transmon qubits [5]. In the latest experiments we investigated different operation regimes for triple quantum dot charge qubits [6, in preparation].
Recent results in the fabrication of bilayer graphene quantum dots [7,8,9,10,11] and the promise of long spin coherence times give rise to new semiconductor based spin qubits. Using state of the art circuit quantum electrodynamics approach we aim to investigate bilayer graphene-based qubits. First proof of principle experiments will be performed with a 50 Ω Aluminum resonator coupled to a plunger gate of a bilayer graphene quantum dot.
References:
[1] A. Stocklauser, et al., PRX, 7 011030 (2017)
[2] A. J. Landig, et al., Nature, 560 179 184 (2018)
[3] D. J. van Woerkom, et al., PRX, 8 041018 (2018)
[4] P. Scarlino, et al., Nature Comm., 10 3011 (2019)
[5] A. J. Landig, et al., Nature Comm., 10 5037 (2019)
[6] J. V. Koski, et al., arXiv 1905.00846 (2019)
[7] H. Overweg, et al. Nano Lett., 18. 553 (2018)
[8] M. Eich, et al., Nano Lett., 18 (8). 5042 (2018)
[9] M. Eich, et al., Phys. Rev. X 8. 031023 (2018)
[10] A. Kurzmann, et al., Nano Lett., 19 (8) 5216 5221 (2019)
[11] A. Kurzmann, et al., PRL, 123 (2) 026803 (2019)
Scanning probe experiments
(Carolin Gold, Lev Ginzburg)
In our group we use two setups to perform scanning gate microscopy at millikelvin-temperatures. This measurement technique uses a voltage-biased scanning probe tip as a mobile, local perturbation. During the measurements, the tip is scanned at constant height to the sample surface while recording the non-local conductance through the sample. Our main topics of investigations are ballistic electron flow and the fractional quantum Hall effect on high mobility GaAs materials. Also different materials, such as graphene, are investigated.